Nihonium
General properties | |||||||||||||||||||||||||||||||||||||||||||||||||||||||||||||||||||||||||||||||||||||||||||||||||||||||||||||||||||||||||||||||||||||||||||||||||||||||||||||||||||||||||||||||||||||||||||||||||||||||||||||||||||||||||||||||||||||
---|---|---|---|---|---|---|---|---|---|---|---|---|---|---|---|---|---|---|---|---|---|---|---|---|---|---|---|---|---|---|---|---|---|---|---|---|---|---|---|---|---|---|---|---|---|---|---|---|---|---|---|---|---|---|---|---|---|---|---|---|---|---|---|---|---|---|---|---|---|---|---|---|---|---|---|---|---|---|---|---|---|---|---|---|---|---|---|---|---|---|---|---|---|---|---|---|---|---|---|---|---|---|---|---|---|---|---|---|---|---|---|---|---|---|---|---|---|---|---|---|---|---|---|---|---|---|---|---|---|---|---|---|---|---|---|---|---|---|---|---|---|---|---|---|---|---|---|---|---|---|---|---|---|---|---|---|---|---|---|---|---|---|---|---|---|---|---|---|---|---|---|---|---|---|---|---|---|---|---|---|---|---|---|---|---|---|---|---|---|---|---|---|---|---|---|---|---|---|---|---|---|---|---|---|---|---|---|---|---|---|---|---|---|---|---|---|---|---|---|---|---|---|---|---|---|---|---|---|---|
Name, symbol | nihonium, Nh | ||||||||||||||||||||||||||||||||||||||||||||||||||||||||||||||||||||||||||||||||||||||||||||||||||||||||||||||||||||||||||||||||||||||||||||||||||||||||||||||||||||||||||||||||||||||||||||||||||||||||||||||||||||||||||||||||||||
Pronunciation |
/nɪˈhoʊniəm/ ni-HOH-nee-əm | ||||||||||||||||||||||||||||||||||||||||||||||||||||||||||||||||||||||||||||||||||||||||||||||||||||||||||||||||||||||||||||||||||||||||||||||||||||||||||||||||||||||||||||||||||||||||||||||||||||||||||||||||||||||||||||||||||||
Nihonium in the periodic table | |||||||||||||||||||||||||||||||||||||||||||||||||||||||||||||||||||||||||||||||||||||||||||||||||||||||||||||||||||||||||||||||||||||||||||||||||||||||||||||||||||||||||||||||||||||||||||||||||||||||||||||||||||||||||||||||||||||
| |||||||||||||||||||||||||||||||||||||||||||||||||||||||||||||||||||||||||||||||||||||||||||||||||||||||||||||||||||||||||||||||||||||||||||||||||||||||||||||||||||||||||||||||||||||||||||||||||||||||||||||||||||||||||||||||||||||
Atomic number (Z) | 113 | ||||||||||||||||||||||||||||||||||||||||||||||||||||||||||||||||||||||||||||||||||||||||||||||||||||||||||||||||||||||||||||||||||||||||||||||||||||||||||||||||||||||||||||||||||||||||||||||||||||||||||||||||||||||||||||||||||||
Group, block | group 13, p-block | ||||||||||||||||||||||||||||||||||||||||||||||||||||||||||||||||||||||||||||||||||||||||||||||||||||||||||||||||||||||||||||||||||||||||||||||||||||||||||||||||||||||||||||||||||||||||||||||||||||||||||||||||||||||||||||||||||||
Period | period 7 | ||||||||||||||||||||||||||||||||||||||||||||||||||||||||||||||||||||||||||||||||||||||||||||||||||||||||||||||||||||||||||||||||||||||||||||||||||||||||||||||||||||||||||||||||||||||||||||||||||||||||||||||||||||||||||||||||||||
Element category | unknown, but probably a post-transition metal | ||||||||||||||||||||||||||||||||||||||||||||||||||||||||||||||||||||||||||||||||||||||||||||||||||||||||||||||||||||||||||||||||||||||||||||||||||||||||||||||||||||||||||||||||||||||||||||||||||||||||||||||||||||||||||||||||||||
Standard atomic weight (Ar) | [286] | ||||||||||||||||||||||||||||||||||||||||||||||||||||||||||||||||||||||||||||||||||||||||||||||||||||||||||||||||||||||||||||||||||||||||||||||||||||||||||||||||||||||||||||||||||||||||||||||||||||||||||||||||||||||||||||||||||||
Electron configuration | [Rn] 5f14 6d10 7s2 7p1 (predicted)[1] | ||||||||||||||||||||||||||||||||||||||||||||||||||||||||||||||||||||||||||||||||||||||||||||||||||||||||||||||||||||||||||||||||||||||||||||||||||||||||||||||||||||||||||||||||||||||||||||||||||||||||||||||||||||||||||||||||||||
per shell | 2, 8, 18, 32, 32, 18, 3 (predicted) | ||||||||||||||||||||||||||||||||||||||||||||||||||||||||||||||||||||||||||||||||||||||||||||||||||||||||||||||||||||||||||||||||||||||||||||||||||||||||||||||||||||||||||||||||||||||||||||||||||||||||||||||||||||||||||||||||||||
Physical properties | |||||||||||||||||||||||||||||||||||||||||||||||||||||||||||||||||||||||||||||||||||||||||||||||||||||||||||||||||||||||||||||||||||||||||||||||||||||||||||||||||||||||||||||||||||||||||||||||||||||||||||||||||||||||||||||||||||||
Phase | solid (predicted)[1][2][3] | ||||||||||||||||||||||||||||||||||||||||||||||||||||||||||||||||||||||||||||||||||||||||||||||||||||||||||||||||||||||||||||||||||||||||||||||||||||||||||||||||||||||||||||||||||||||||||||||||||||||||||||||||||||||||||||||||||||
Melting point | 700 K (430 °C, 810 °F) (predicted)[1] | ||||||||||||||||||||||||||||||||||||||||||||||||||||||||||||||||||||||||||||||||||||||||||||||||||||||||||||||||||||||||||||||||||||||||||||||||||||||||||||||||||||||||||||||||||||||||||||||||||||||||||||||||||||||||||||||||||||
Boiling point | 1430 K (1130 °C, 2070 °F) (predicted)[1][4] | ||||||||||||||||||||||||||||||||||||||||||||||||||||||||||||||||||||||||||||||||||||||||||||||||||||||||||||||||||||||||||||||||||||||||||||||||||||||||||||||||||||||||||||||||||||||||||||||||||||||||||||||||||||||||||||||||||||
Density near r.t. | 16 g/cm3 (predicted)[4] | ||||||||||||||||||||||||||||||||||||||||||||||||||||||||||||||||||||||||||||||||||||||||||||||||||||||||||||||||||||||||||||||||||||||||||||||||||||||||||||||||||||||||||||||||||||||||||||||||||||||||||||||||||||||||||||||||||||
Heat of fusion | 7.61 kJ/mol (extrapolated)[3] | ||||||||||||||||||||||||||||||||||||||||||||||||||||||||||||||||||||||||||||||||||||||||||||||||||||||||||||||||||||||||||||||||||||||||||||||||||||||||||||||||||||||||||||||||||||||||||||||||||||||||||||||||||||||||||||||||||||
Heat of vaporization | 130 kJ/mol (predicted)[2][4] | ||||||||||||||||||||||||||||||||||||||||||||||||||||||||||||||||||||||||||||||||||||||||||||||||||||||||||||||||||||||||||||||||||||||||||||||||||||||||||||||||||||||||||||||||||||||||||||||||||||||||||||||||||||||||||||||||||||
Atomic properties | |||||||||||||||||||||||||||||||||||||||||||||||||||||||||||||||||||||||||||||||||||||||||||||||||||||||||||||||||||||||||||||||||||||||||||||||||||||||||||||||||||||||||||||||||||||||||||||||||||||||||||||||||||||||||||||||||||||
Oxidation states | −1, 1, 3, 5 (predicted)[1][4][5] | ||||||||||||||||||||||||||||||||||||||||||||||||||||||||||||||||||||||||||||||||||||||||||||||||||||||||||||||||||||||||||||||||||||||||||||||||||||||||||||||||||||||||||||||||||||||||||||||||||||||||||||||||||||||||||||||||||||
Ionization energies |
1st: 704.9 kJ/mol (predicted)[1] 2nd: 2238.5 kJ/mol (predicted)[4] 3rd: 3203.3 kJ/mol (predicted)[4] (more) | ||||||||||||||||||||||||||||||||||||||||||||||||||||||||||||||||||||||||||||||||||||||||||||||||||||||||||||||||||||||||||||||||||||||||||||||||||||||||||||||||||||||||||||||||||||||||||||||||||||||||||||||||||||||||||||||||||||
Atomic radius | empirical: 170 pm (predicted)[1] | ||||||||||||||||||||||||||||||||||||||||||||||||||||||||||||||||||||||||||||||||||||||||||||||||||||||||||||||||||||||||||||||||||||||||||||||||||||||||||||||||||||||||||||||||||||||||||||||||||||||||||||||||||||||||||||||||||||
Covalent radius | 172–180 pm (extrapolated)[3] | ||||||||||||||||||||||||||||||||||||||||||||||||||||||||||||||||||||||||||||||||||||||||||||||||||||||||||||||||||||||||||||||||||||||||||||||||||||||||||||||||||||||||||||||||||||||||||||||||||||||||||||||||||||||||||||||||||||
Miscellanea | |||||||||||||||||||||||||||||||||||||||||||||||||||||||||||||||||||||||||||||||||||||||||||||||||||||||||||||||||||||||||||||||||||||||||||||||||||||||||||||||||||||||||||||||||||||||||||||||||||||||||||||||||||||||||||||||||||||
CAS Number | 54084-70-7 | ||||||||||||||||||||||||||||||||||||||||||||||||||||||||||||||||||||||||||||||||||||||||||||||||||||||||||||||||||||||||||||||||||||||||||||||||||||||||||||||||||||||||||||||||||||||||||||||||||||||||||||||||||||||||||||||||||||
History | |||||||||||||||||||||||||||||||||||||||||||||||||||||||||||||||||||||||||||||||||||||||||||||||||||||||||||||||||||||||||||||||||||||||||||||||||||||||||||||||||||||||||||||||||||||||||||||||||||||||||||||||||||||||||||||||||||||
Naming | After Japan (Nihon in Japanese) | ||||||||||||||||||||||||||||||||||||||||||||||||||||||||||||||||||||||||||||||||||||||||||||||||||||||||||||||||||||||||||||||||||||||||||||||||||||||||||||||||||||||||||||||||||||||||||||||||||||||||||||||||||||||||||||||||||||
Discovery |
RIKEN (2004, first undisputed) Joint Institute for Nuclear Research and Lawrence Livermore National Laboratory (2003, first announced) | ||||||||||||||||||||||||||||||||||||||||||||||||||||||||||||||||||||||||||||||||||||||||||||||||||||||||||||||||||||||||||||||||||||||||||||||||||||||||||||||||||||||||||||||||||||||||||||||||||||||||||||||||||||||||||||||||||||
Most stable isotopes of nihonium | |||||||||||||||||||||||||||||||||||||||||||||||||||||||||||||||||||||||||||||||||||||||||||||||||||||||||||||||||||||||||||||||||||||||||||||||||||||||||||||||||||||||||||||||||||||||||||||||||||||||||||||||||||||||||||||||||||||
| |||||||||||||||||||||||||||||||||||||||||||||||||||||||||||||||||||||||||||||||||||||||||||||||||||||||||||||||||||||||||||||||||||||||||||||||||||||||||||||||||||||||||||||||||||||||||||||||||||||||||||||||||||||||||||||||||||||
Nihonium (symbol Nh) is a chemical element with atomic number 113. It is a synthetic element (an element that can be created in a laboratory but is not found in nature) and is extremely radioactive; its most stable known isotope, nihonium-286, has a half-life of 20 seconds. It is also known as eka-thallium or simply element 113. Nihonium was first reported to have been created in 2003 by the Joint Institute for Nuclear Research in Dubna, Russia, and in 2004 by a team of Japanese scientists at RIKEN. In December 2015, the International Union of Pure and Applied Chemistry (IUPAC) and the International Union of Pure and Applied Physics (IUPAP) recognized the element and assigned the priority of the discovery to RIKEN.[6] In November 2016, the IUPAC published a declaration defining the name to be nihonium.[7] The name comes from the common Japanese name for Japan (日本 nihon). On 28 November 2016, the name became official.[8][9]
In the periodic table, it is a p-block transactinide element. It is a member of the 7th period and is placed in the boron group, although it has not been confirmed to behave as the heavier homologue to thallium in the boron group. Nihonium is calculated to have some similar properties to its lighter homologues, boron, aluminium, gallium, indium, and thallium, and behave as a post-transition metal, although it should also show several major differences from them. Unlike all the other p-block elements, it may be able to involve its d-electrons in bonding, although these predictions are disputed.
History
Dubna–Livermore collaboration
The first report of nihonium was in August 2003, when it was identified as an alpha decay product of element 115, moscovium. These results were published on February 1, 2004, by a team composed of Russian scientists at Dubna (Joint Institute for Nuclear Research), and American scientists at the Lawrence Livermore National Laboratory:[10][11]
- 243
95Am
+ 48
20Ca
→ 288
115Mc
+ 3 1
0n
→ 284
113Nh
+
α - 243
95Am
+ 48
20Ca
→ 287
115Mc
+ 4 1
0n
→ 283
113Nh
+
α
The Dubna–Livermore collaboration has strengthened their claim for the discovery of nihonium by conducting chemical experiments on 268Db, the final decay product of 288Mc. This was valuable as none of the nuclides in this decay chain were previously known, so that their claim was not supported by any previously obtained experimental data (as none existed), and chemical experimentation would strengthen the case for their claim. In June 2004 and again in December 2005, this dubnium isotope was successfully identified by extracting the final decay products, measuring spontaneous fission (SF) activities and using chemical identification techniques to confirm that they behave like a group 5 element (as dubnium is known to be in group 5 of the periodic table).[1][12] Both the half-life and decay mode were confirmed for the proposed 268Db which lends support to the assignment of the parent and daughter nuclei to moscovium and nihonium respectively.[12][13] Further experiments at Dubna in 2005 have fully confirmed the decay data for moscovium and nihonium, but in 2011, the IUPAC/IUPAP Joint Working Party (JWP) did not recognize the two elements as having been discovered because current theory could not distinguish between group 4 and group 5 elements by their chemical properties with sufficient confidence, and the identification of the daughter dubnium isotope was the most important factor in confirming the discovery of moscovium and nihonium.[14] Furthermore, the decay properties of all the nuclei in the decay chain of moscovium had not been previously characterized before the Dubna experiments, a situation which the JWP generally considers "troublesome, but not necessarily exclusive".[14]
RIKEN
On July 23, 2004, a team of Japanese scientists at RIKEN bombarded a target of bismuth-209 with accelerated nuclei of zinc-70 and detected a single atom of the isotope nihonium-278. They published their results on September 28, 2004:[15]
Previously, in 2000, a team led by P. A. Wilk identified the decay product 266Bh as decaying with identical properties to what the Japanese team had observed, thus lending support for their claim. However, they also observed the daughter of 266Bh, 262Db, undergo alpha decay instead of spontaneous fission (the Japanese team observed the latter decay mode).[14]
The RIKEN team produced a further atom on April 2, 2005, although the decay data were slightly different from the first chain, perhaps due to either the formation of a metastable state or an alpha particle escaping from the detector before depositing its full energy. Due to these inconsistencies in the decay data, the small number of nihonium atoms produced, and the lack of unambiguous anchors to known isotopes, the JWP did not accept this as a conclusive discovery of nihonium in 2011.[14]
Most recently, production and identification of another 278Nh nucleus occurred at RIKEN on August 12, 2012.[16][17] In this case, a series of six alpha decays was observed, leading down to an isotope of mendelevium:
This decay chain differed from the previous observations at RIKEN mainly in the decay mode of dubnium, which was previously observed to undergo spontaneous fission, but in this case instead alpha decayed; the alpha decay of 262Db to 258Lr is well-known. The scientists on this team calculated the probability of accidental coincidence to be 10−28, or totally negligible.[16] The resulting 254Md atom than underwent beta plus decay to 254Fm, which itself finally alpha decayed to the long-lived 250Cf, which has a half-life of around thirteen years.[18]
Naming
Using Mendeleev's nomenclature for unnamed and undiscovered elements, nihonium should be known as eka-thallium. In 1979 IUPAC published recommendations according to which the element was to be called ununtrium (with the corresponding symbol of Uut),[19] a systematic element name as a placeholder, until the discovery of the element is confirmed and a name is decided on. Although widely used in the chemical community on all levels, from chemistry classrooms to advanced textbooks, the recommendations were mostly ignored among scientists in the field, who call it "element 113", with the symbol of (113) or even simply 113.[1]
Claims to the discovery of nihonium were put forward by both the Dubna and RIKEN teams. The Japanese team suggested various names: japonium, symbol Jp, after their home country;[20] nishinanium, symbol Nh, after Japanese physicist Yoshio Nishina, the "founding father of modern physics research in Japan";[21] and rikenium, symbol Rk, after the team itself.[20] In 2011, the IUPAC evaluated the 2004 RIKEN experiments and 2004 and 2007 Dubna experiments, and concluded that they did not meet the criteria for discovery.[14]
On August 12, 2012, researchers at the RIKEN Nishina Center for Accelerator-Based Science in Japan, claimed to have synthesised element 113 by colliding zinc nuclei (with 30 protons each) into a thin layer of bismuth (which contains 83 protons).[22] In December 2015, IUPAC recognized the element and assigned the priority of the discovery to RIKEN.[23] For the first time in history a team of Asian physicists will name a new element.[22] In March 2016, Kosuke Morita, the leader of the RIKEN team, proposed the name "nihonium" to IUPAC, after its place of discovery and referencing Japanese chemist Masataka Ogawa's 1908 discovery of rhenium, which he named "nipponium".[23] The IUPAC has accepted the proposal[24] and set a term expiring 8 November to collect comments, after which the final name will be formally established at a conference.[25][26]
![]() |
Wikinews has related news: IUPAC proposes four new chemical element names |
On 8 June 2016, IUPAC disclosed the name of element 113 as nihonium. Prior to the formal approval by the IUPAC Council, a five-month public review was set, expiring 8 November 2016; the name was officially approved on 28 November 2016.
Isotopes
Isotope | Half-life [27] | Decay mode[27] | Discovery year | Reaction |
---|---|---|---|---|
278Nh | 0.24 ms | α | 2004 | 209Bi(70Zn,n)[15] |
282Nh | 70 ms | α | 2006 | 237Np(48Ca,3n)[28] |
283Nh | 0.10 s | α | 2003 | 287Mc(—,α)[28] |
284Nh | 0.48 s | α, EC | 2003 | 288Mc(—,α)[28] |
285Nh | 5.5 s | α | 2009 | 293Ts(—,2α)[29] |
286Nh | 20 s | α | 2009 | 294Ts(—,2α)[29] |
287Nh | 20? min | α, SF ? | unknown | — |
Nihonium has no stable or naturally occurring isotopes. Several radioactive isotopes have been synthesized in the laboratory, either by fusing two atoms or by observing the decay of heavier elements. Six different isotopes of nihonium have been reported with atomic masses 278 and 282–286; they all decay through alpha decay,[27] although nihonium-284 may have an electron capture branch.[30]
Stability and half-lives

All nihonium isotopes are extremely unstable and radioactive; however, the heavier nihonium isotopes are more stable than the lighter. The most stable known nihonium isotope, 286Nh, is also the heaviest known nihonium isotope; it has a half-life of 20 seconds. The isotope 285Nh has been reported to also have a half-life of over a second. The isotopes 284Nh and 283Nh have half-lives of 0.48 and 0.10 seconds respectively. The remaining two isotopes have half-lives between 0.1 and 100 milliseconds: 282Nh has a half-life of 70 milliseconds, and 278Nh, the lightest known nihonium isotope, is also the shortest-lived known nihonium isotope, with a half-life of just 0.24 milliseconds. It is predicted that even heavier undiscovered nihonium isotopes could be much more stable: for example, 287Nh is predicted to have a half-life of around 20 minutes,[31] close to two orders of magnitude more than that of 286Nh.[27]
Theoretical estimates of alpha decay half-lives of isotopes of nihonium are in good agreement with the experimental data.[32] The undiscovered isotope 293Nh has been predicted to be the most stable towards beta decay;[33] however, no known nihonium isotope has been observed to undergo beta decay.[27]
The stability of nuclei decreases greatly with the increase in atomic number after plutonium, the heaviest primordial element, so that all isotopes with an atomic number above 101 decay radioactively with a half-life under a day, with the exception of dubnium-268. Nevertheless, because of reasons not very well understood yet, there is a slight increased nuclear stability around atomic numbers 110–114, which leads to the appearance of what is known in nuclear physics as the "island of stability". This concept, proposed by University of California professor Glenn Seaborg, explains why superheavy elements last longer than predicted.[34]
Predicted properties
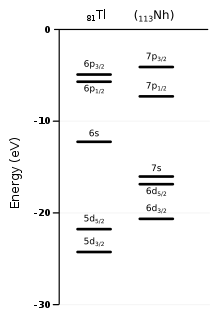
Nihonium is the first member of the 7p series of elements and the heaviest boron group element on the periodic table, below boron, aluminium, gallium, indium, and thallium. It is predicted to show many differences from its lighter homologues: a largely contributing effect is the spin–orbit (SO) interaction. It is especially strong for the superheavy elements, because their electrons move much faster than in lighter atoms, at velocities comparable to the speed of light.[36] In relation to nihonium atoms, it lowers the 7s and the 7p electron energy levels (stabilizing the corresponding electrons), but two of the 7p electron energy levels are stabilized more than the other four.[37] The stabilization of the 7s electrons is called the inert pair effect, and the effect "tearing" the 7p subshell into the more stabilized and the less stabilized parts is called the subshell splitting. Computation chemists see the split as a change of the second (azimuthal) quantum number l from 1 to 1/2 and 3/2 for the more stabilized and less stabilized parts of the 7p subshell, respectively.[36][note 1] For many theoretical purposes, the valence electron configuration may be represented to reflect the 7p subshell split as 7s27p1/21.[1] These effects stabilize lower oxidation states: the first ionization energy of nihonium is expected to be 7.306 eV, the highest among the boron group elements. Hence, the most stable oxidation state of nihonium is predicted to be the +1 state,[1] and nihonium is expected to be less reactive than thallium.[4] Differences for other electron levels also exist. For example, the 6d electron levels (also split in halves, with four being 6d3/2 and six being 6d5/2) are both raised, so that they are close in energy to the 7s ones.[37] Thus, the 6d electron levels, being destabilized, should still be able to participate in chemical reactions in nihonium[1] (as well as in the next 7p element, flerovium),[4] thus making it behave in some ways like transition metals and allow higher oxidation states.[1] Nihonium should hence also be able to show stable +3 and possibly also +5 oxidation states. However, the +3 state should still be less stable than the +1 state, following periodic trends. Nihonium should be the most electronegative among all the boron group elements:[1] for example, in the compound NhTs, the negative charge is expected to be on the nihonium atom rather than the tennessine atom, the opposite of what would be expected from simple periodicity.[35] The electron affinity of nihonium is calculated to be around 0.68 eV; in comparison, that of thallium is 0.4 eV.[1] The high electron affinity and electronegativity of nihonium are due to it being only one electron short of the closed-shell valence electron configuration of flerovium (7s27p1/22):[1] this would make the −1 oxidation state of nihoniummore stable than that of its lighter congener thallium.[5] The standard electrode potential for the Nh+/Nh couple is predicted to be −0.6 V.[4]
The simplest possible nihonium compound is the monohydride, NhH. The bonding is provided by the 7p1/2 electron of nihonium and the 1s electron of hydrogen. However, the SO interaction causes the binding energy of nihonium monohydride to be reduced by about 1 eV[1] and the nihonium–hydrogen bond length to decrease as the bonding 7p1/2 orbital is relativistically contracted. This is exceptional in the 7p series of elements; all other MH (M = Fl, Mc, Lv, Ts, Og) molecules have relativistic expansion of the bond length instead of contraction.[38] The analogous monofluoride (NhF) should also exist.[35] Nihonium should also be able to form the trihydride (NhH3), trifluoride (NhF3), and trichloride (NhCl3), with nihonium in the +3 oxidation state. Because the 6d electrons are involved in bonding instead of the 7s ones, these molecules are predicted to be T-shaped and not trigonal planar. Although the polyfluoride anion NhF−
6 should be stable, the corresponding neutral fluoride NhF5 should be unstable, spontaneously decomposing into the trifluoride and elemental fluorine. Nihonium(I) is predicted to be more similar to silver(I) than thallium(I):[1] the Nh+ ion is expected to more willingly bind anions, so that NhCl should be quite soluble in an excess of hydrochloric acid or in ammonia while TlCl is not. Additionally, in contrast to the strongly basic TlOH, nihonium(I) should instead form Nh2O, which would be weakly water-soluble and readily ammonia-soluble.[4]
Nihonium is expected to be much denser than thallium, having a predicted density of about 16 to 18 g/cm3, due to the relativistic stabilization and contraction of its 7s and 7p1/2 orbitals.[1][35] This is because calculations estimate it to have an atomic radius of about 170 pm, the same as that of thallium, even though periodic trends would predict it to have an atomic radius larger than that of thallium due to it being one period further down in the periodic table.[1] The melting and boiling points of nihonium are not definitely known, but have been calculated to be 430 °C and 1100 °C respectively, exceeding the values for gallium, indium, and thallium, following periodic trends.[1][2]
Experimental chemistry
Unambiguous determination of the chemical characteristics of nihonium has yet to have been established.[39][40] The isotopes 284Nh, 285Nh, and 286Nh have half-lives long enough for chemical investigation. It is theoretically predicted that nihonium should have an enthalpy of sublimation around 150 kJ/mol and an enthalpy of adsorption on a gold surface around −159 kJ/mol.[40] From 2010 to 2012, some preliminary chemical experiments were performed to determine the volatility of nihonium. The reaction used was 243Am(48Ca,3n)288Mc; the isotope 288Mc has a short half-life and would quickly decay to the longer-lived 284Nh, which would be chemically investigated. Teflon capillaries at 70 °C connecting the recoil chamber, where the nihonium atoms were synthesized, and the gold-covered detectors: the nihonium atoms would be carried along the capillaries by a carrier gas. While about ten to twenty atoms of 284Nh were produced, none of these atoms were registered by the gold-covered detectors, suggesting either that nihonium was similar in volatility to the noble gases or, more plausibly, that pure nihonium was not very volatile and thus could not efficiently pass through the Teflon capillaries at 70 °C.[40] Formation of the hydroxide NhOH would ease the transport, as NhOH is expected to be more volatile than elemental nihonium, and this reaction could be facilitated by adding more water vapor into the carrier gas. However, it seems likely that this formation is not kinetically favored, so that one would need to use the longer-lived isotope 286Nh in future experiments.[40]
See also
Notes
- ↑ The quantum number corresponds to the letter in the electron orbital name: 0 to s, 1 to p, 2 to d, etc. See azimuthal quantum number for more information.
References
- 1 2 3 4 5 6 7 8 9 10 11 12 13 14 15 16 17 18 19 20 21 Hoffman, Darleane C.; Lee, Diana M.; Pershina, Valeria (2006). "Transactinides and the future elements". In Morss; Edelstein, Norman M.; Fuger, Jean. The Chemistry of the Actinide and Transactinide Elements (3rd ed.). Dordrecht, The Netherlands: Springer Science+Business Media. ISBN 1-4020-3555-1.
- 1 2 3 Seaborg, Glenn T. (c. 2006). "transuranium element (chemical element)". Encyclopædia Britannica. Retrieved 2010-03-16.
- 1 2 3 Bonchev, Danail; Kamenska, Verginia (1981). "Predicting the Properties of the 113–120 Transactinide Elements". Journal of Physical Chemistry. American Chemical Society. 85 (9): 1177–1186. doi:10.1021/j150609a021.
- 1 2 3 4 5 6 7 8 9 10 Fricke, Burkhard (1975). "Superheavy elements: a prediction of their chemical and physical properties". Recent Impact of Physics on Inorganic Chemistry. 21: 89–144. doi:10.1007/BFb0116498. Retrieved 4 October 2013.
- 1 2 Thayer, John S. (2010). "Relativistic Effects and the Chemistry of the Heavier Main Group Elements": 82. doi:10.1007/978-1-4020-9975-5_2.
- ↑ IUPAC (2015). "Discovery and Assignment of Elements with Atomic Numbers 113, 115, 117 and 118". Retrieved 2016-01-04.
- ↑ "IUPAC Announces the Names of the Elements 113, 115, 117, and 118". IUPAC. 2016-11-30. Retrieved 2016-11-30.
- ↑ Staff (30 November 2016). "IUPAC Announces the Names of the Elements 113, 115, 117, and 118". IUPAC. Retrieved 1 December 2016.
- ↑ St. Fleur, Nicholas (1 December 2016). "Four New Names Officially Added to the Periodic Table of Elements". New York Times. Retrieved 1 December 2016.
- ↑ "Experiments on the synthesis of element 115 in the reaction 243Am(48Ca,xn)291-x115", Oganessian et al., JINR Preprints, 2003. Retrieved on 3 March 2008
- ↑ Oganessian, Yu. Ts.; Utyonkoy, V.; Lobanov, Yu.; Abdullin, F.; Polyakov, A.; Shirokovsky, I.; Tsyganov, Yu.; Gulbekian, G.; Bogomolov, S.; Mezentsev, A. N.; et al. (2004). "Experiments on the synthesis of element 115 in the reaction 243Am(48Ca,xn)291-x115". Physical Review C. 69 (2): 021601. Bibcode:2004PhRvC..69b1601O. doi:10.1103/PhysRevC.69.021601.
- 1 2 "Results of the experiment on chemical identification of Db as a decay product of element 115", Oganessian et al., JINR preprints, 2004. Retrieved on 3 March 2008
- ↑ Oganessian, Yu. Ts.; Utyonkov, V.; Dmitriev, S.; Lobanov, Yu.; Itkis, M.; Polyakov, A.; Tsyganov, Yu.; Mezentsev, A.; Yeremin, A.; Voinov, A. A.; et al. (2005). "Synthesis of elements 115 and 113 in the reaction 243Am + 48Ca". Physical Review C. 72 (3): 034611. Bibcode:2005PhRvC..72c4611O. doi:10.1103/PhysRevC.72.034611.
- 1 2 3 4 5 Barber, Robert C.; Karol, Paul J; Nakahara, Hiromichi; Vardaci, Emanuele; Vogt, Erich W. (2011). "Discovery of the elements with atomic numbers greater than or equal to 113 (IUPAC Technical Report)". Pure Appl. Chem. 83 (7): 1485. doi:10.1351/PAC-REP-10-05-01.
- 1 2 Morita, Kosuke; Morimoto, Kouji; Kaji, Daiya; Akiyama, Takahiro; Goto, Sin-ichi; Haba, Hiromitsu; Ideguchi, Eiji; Kanungo, Rituparna; Katori, Kenji; Koura, Hiroyuki; Kudo, Hisaaki; Ohnishi, Tetsuya; Ozawa, Akira; Suda, Toshimi; Sueki, Keisuke; Xu, HuShan; Yamaguchi, Takayuki; Yoneda, Akira; Yoshida, Atsushi; Zhao, YuLiang (2004). "Experiment on the Synthesis of Element 113 in the Reaction 209Bi(70Zn,n)278113". Journal of the Physical Society of Japan. 73 (10): 2593–2596. Bibcode:2004JPSJ...73.2593M. doi:10.1143/JPSJ.73.2593.
- 1 2 K. Morita; Morimoto, Kouji; Kaji, Daiya; Haba, Hiromitsu; Ozeki, Kazutaka; Kudou, Yuki; Sumita, Takayuki; Wakabayashi, Yasuo; Yoneda, Akira; Tanaka, Kengo; et al. (2012). "New Results in the Production and Decay of an Isotope, 278113, of the 113th Element". Journal of the Physical Society of Japan. 81 (10): 103201. arXiv:1209.6431
. Bibcode:2012JPSJ...81j3201M. doi:10.1143/JPSJ.81.103201.
- ↑ "Search for element 113 concluded at last". Press Release. RIKEN. 27 September 2012. Retrieved 27 September 2012.
- ↑ SHE Research at RIKEN/GARIS by Kosuke Morita
- ↑ Chatt, J. (1979). "Recommendations for the Naming of Elements of Atomic Numbers Greater than 100". Pure Appl. Chem. 51 (2): 381–384. doi:10.1351/pac197951020381.
- 1 2 Noorden, Richard Van. "Element 113 at Last?".
- ↑ 新元素113番、日本の発見確実に 合成に3回成功. Nihon Keizai Shimbun (in Japanese). 2012-09-27. Retrieved 2012-10-13.
- 1 2 "Element 113: Ununtrium Reportedly Synthesised In Japan". Huffington Post. September 2012. Retrieved 22 April 2013.
- 1 2 Discovery and Assignment of Elements with Atomic Numbers 113, 115, 117 and 118. IUPAC (2015-12-30)
- ↑ "IUPAC Is Naming The Four New Elements Nihonium, Moscovium, Tennessine, And Oganesson". IUPAC. 2016-06-08. Retrieved 2016-06-08.
- ↑ "Japan scientists plan to name atomic element 113 'Nihonium'". Mainichi Shimbun. June 8, 2016.
Japanese scientists who discovered the atomic element 113 plan to name it "Nihonium", sources close to the matter said Wednesday.
- ↑ "ニホニウム」有力 日本初の新元素名称案、国際機関が9日公表" [Nihonium the most probable] (in Japanese). The Sankei Shimbun. June 6, 2016.
Rather than initially proposed Japanium which is derived from Latin or French, Morita group leader seems to stick to his own language.
- 1 2 3 4 5 Sonzogni, Alejandro. "Interactive Chart of Nuclides". National Nuclear Data Center: Brookhaven National Laboratory. Retrieved 2008-06-06.
- 1 2 3 Oganessian, Yu. Ts.; Penionzhkevich, Yu. E.; Cherepanov, E. A. (2007). "Heaviest Nuclei Produced in 48Ca-induced Reactions (Synthesis and Decay Properties)". AIP Conference Proceedings. 912: 235. doi:10.1063/1.2746600.
- 1 2 Oganessian, Yuri Ts.; Abdullin, F. Sh.; Bailey, P. D.; et al. (2010-04-09). "Synthesis of a New Element with Atomic Number Z=117". Physical Review Letters. American Physical Society. 104 (142502). Bibcode:2010PhRvL.104n2502O. doi:10.1103/PhysRevLett.104.142502. PMID 20481935.
- ↑ http://xxx.lanl.gov/pdf/1502.03030.pdf
- ↑ Audi, Georges; Bersillon, O.; Blachot, J.; Wapstra, A.H. (2003). "The NUBASE Evaluation of Nuclear and Decay Properties". Nuclear Physics A. Atomic Mass Data Center. 729: 3–128. Bibcode:2003NuPhA.729....3A. doi:10.1016/j.nuclphysa.2003.11.001.
- ↑ Chowdhury, P. Roy; Basu, D. N. & Samanta, C. (2007). "α decay chains from element 113". Phys. Rev. C. 75 (4): 047306. arXiv:0704.3927
. Bibcode:2007PhRvC..75d7306C. doi:10.1103/PhysRevC.75.047306.
- ↑ Nie, G. K. (2005). "Charge radii of β-stable nuclei". Modern Physics Letters A. 21 (24): 1889. arXiv:nucl-th/0512023
. Bibcode:2006MPLA...21.1889N. doi:10.1142/S0217732306020226.
- ↑ Considine, Glenn D.; Kulik, Peter H. (2002). Van Nostrand's scientific encyclopedia (9th ed.). Wiley-Interscience. ISBN 978-0-471-33230-5. OCLC 223349096.
- 1 2 3 4 Stysziński, Jacek (2010). Why do we Need Relativistic Computational Methods?. pp. 139–146. doi:10.1007/9781402099755_3.
- 1 2 Thayer, John S. (2010). "Relativistic Effects and the Chemistry of the Heavier Main Group Elements": 63–67. doi:10.1007/978-1-4020-9975-5_2.
- 1 2 Fægri Jr., Knut; Saue, Trond (2001). "Diatomic molecules between very heavy elements of group 13 and group 17: A study of relativistic effects on bonding". The Journal of Chemical Physics. American Institute of Physics. 115 (6): 2456. Bibcode:2001JChPh.115.2456F. doi:10.1063/1.1385366.
- ↑ Han, Young-Kyu; Bae, Cheolbeom; Son, Sang-Kil; Lee, Yoon Sup (2000). "Spin–orbit effects on the transactinide p-block element monohydrides MH (M=element 113–118)". Journal of Chemical Physics. 112 (6): 2684. Bibcode:2000JChPh.112.2684H. doi:10.1063/1.480842.
- ↑ Düllmann, Christoph E. (2012). "Superheavy elements at GSI: a broad research program with element 114 in the focus of physics and chemistry". Radiochimica Acta. 100 (2): 67–74. doi:10.1524/ract.2011.1842.
- 1 2 3 4 Eichler, Robert (2013). "First foot prints of chemistry on the shore of the Island of Superheavy Elements" (PDF). Journal of Physics: Conference Series. IOP Science. 420 (1). doi:10.1088/1742-6596/420/1/012003. Retrieved 11 September 2014.
External links
- Nihonium at The Periodic Table of Videos (University of Nottingham)
- Uut and Uup Add Their Atomic Mass to Periodic Table
- Discovery of Elements 113 and 115
- Superheavy elements
- WebElements.com: Nihonium
Periodic table (Large cells) | |||||||||||||||||||||||||||||||||
---|---|---|---|---|---|---|---|---|---|---|---|---|---|---|---|---|---|---|---|---|---|---|---|---|---|---|---|---|---|---|---|---|---|
1 | 2 | 3 | 4 | 5 | 6 | 7 | 8 | 9 | 10 | 11 | 12 | 13 | 14 | 15 | 16 | 17 | 18 | ||||||||||||||||
1 | H | He | |||||||||||||||||||||||||||||||
2 | Li | Be | B | C | N | O | F | Ne | |||||||||||||||||||||||||
3 | Na | Mg | Al | Si | P | S | Cl | Ar | |||||||||||||||||||||||||
4 | K | Ca | Sc | Ti | V | Cr | Mn | Fe | Co | Ni | Cu | Zn | Ga | Ge | As | Se | Br | Kr | |||||||||||||||
5 | Rb | Sr | Y | Zr | Nb | Mo | Tc | Ru | Rh | Pd | Ag | Cd | In | Sn | Sb | Te | I | Xe | |||||||||||||||
6 | Cs | Ba | La | Ce | Pr | Nd | Pm | Sm | Eu | Gd | Tb | Dy | Ho | Er | Tm | Yb | Lu | Hf | Ta | W | Re | Os | Ir | Pt | Au | Hg | Tl | Pb | Bi | Po | At | Rn | |
7 | Fr | Ra | Ac | Th | Pa | U | Np | Pu | Am | Cm | Bk | Cf | Es | Fm | Md | No | Lr | Rf | Db | Sg | Bh | Hs | Mt | Ds | Rg | Cn | Nh | Fl | Mc | Lv | Ts | Og | |
|