Sperm guidance
Sperm guidance is the process by which sperm cells (spermatozoa) are directed to the oocyte (egg) for the aim of fertilization. In the case of marine invertebrates the guidance is done by chemotaxis. In the case of mammals, it appears to be done by both chemotaxis and thermotaxis.
Background
Since the discovery of sperm attraction to the female gametes in ferns over a century ago (Pfeffer, 1884), sperm guidance in the form of sperm chemotaxis has been established in a large variety of species (Miller, 1985). Although sperm chemotaxis is prevalent throughout the Metazoa kingdom, from marine species with external fertilization such as sea urchins and corals, to humans (Cosson, 1990; Eisenbach and Tur-Kaspa, 1994; Miller, 1985), most of the current information on sperm chemotaxis is derived from studies of marine invertebrates, primarily sea urchin and starfish (Kaupp et al., 2006). As a matter of fact, until not too long ago, the dogma was that, in mammals, guidance of spermatozoa to the oocyte was unnecessary. This was due to the common belief that, following ejaculation into the female genital tract, large numbers of spermatozoa 'race' towards the oocyte and compete to fertilize it. This belief was taken apart when it became clear that only few of the ejaculated spermatozoa — in humans, only ~1 of every million spermatozoa — succeed in entering the oviducts (Fallopian tubes) [Eisenbach and Tur-Kaspa (1999) for a review], and when more recent studies showed that mammalian spermatozoa employ two different guidance mechanisms: chemotaxis (Ralt et al., 1994) and thermotaxis (Bahat et al., 2003).
Sperm guidance in non-mammalian species
Sperm guidance in non-mammalian species is performed by chemotaxis. The oocyte secretes a chemoattractant, which, as it diffuses away, forms a concentration gradient: a high concentration close to the egg, and a gradually lower concentration as the distance from the oocyte is longer. Spermatozoa can sense this chemoattractant and orient their swimming direction up the concentration gradient towards the oocyte. Sperm chemotaxis was demonstrated in a large number of non-mammalian species, from marine invertebrates (Cosson, 1990; Miller, 1985) to frogs (Al-Anzi and Chandler, 1998).
Chemoattractants
The sperm chemoattractants in non-mammalian species vary to a large extent. Some examples are shown in Table 1. So far, most sperm chemoattractants that have been identified in non-mammalian species are peptides or low-molecular-weight proteins (1–20 kDa), which are heat stable and sensitive to proteases (Cosson, 1990; Miller, 1985). Exceptions to this rule are the sperm chemoattractants of corals, ascidians, plants such as ferns, and algae (Table 1).
Table 1. Some sperm chemoattractants in non-mammalian species*
Species | Chemoattractant | References |
---|---|---|
Algae | Low-molecular-weight unsaturated pheromones of cyclic or linear structure (for example 532 Da pentosylated hydroquinone in the case of Chlamydomonas allensworthii) | (Cosson, 1990; Maier and Müller, 1986; Starr et al., 1995) |
Amphibians | Allurin — a 21 kDa protein (for Xenopus) | (Al-Anzi and Chandler, 1998; Olson et al., 2001) |
Ascidians | SAAF — a sulfated steroid: 3,4,7,26-tetrahydroxycholestane-3,26-disulfate (for Ciona savignyi and intestinalis) | (Yoshida et al., 1993; 1994; 2002) |
Corals | A lipid-like long chain fatty alcohol CH3-(CH2)8-CH=CH-CH=CH-CH2OH (for Montipora digitata) | (Coll and Miller, 1992) |
Ferns | Dicarboxylic acids, for example malic acid in its partially ionized form (for Pteridium aquilinum) | (Brokaw, 1958) |
Mollusks | SepSAP — a 6-residue peptide-amide with the sequence PIDPGV-CONH2 (for Sepia officinalis) | (Zatylny et al., 2002) |
Sea urchins | Resact — a 14-residue peptide with the sequence CVTGAPGCVGGGRL-NH2 (for Arbacia punctulata) | (Ward et al., 1985) |
Starfish | Startrak — a 13 kDa heat-stable protein (for Pycnopodia helianthoides) | (Miller and Vogt, 1996) |
- Taken from (Eisenbach, 2004).
Species specificity
The variety of chemoattractants raises the question of species specificity with respect to the identity of chemoattractant. There is no single rule for chemoattractant-related specificity. Thus, in some groups of marine invertebrates (e.g., hydromedusae and certain ophiuroids), the specificity is very high; in others (e.g., starfish), the specificity is at the family level and, within the family, there is no specificity (Cosson, 1990; Miller, 1985, 1997). In mollusks, there appears to be no specificity at all. Likewise, in plants, a unique simple compound [e.g., fucoserratene — a linear, unsaturated alkene (1,3-trans 5-cis-octatriene)] might be a chemoattractant for various species (Maier and Müller, 1986).
Behavioral mechanism
Here, too, there is no single rule. In some species (for example, in hydroids like Campanularia or tunicate like Ciona), the swimming direction of the spermatozoa changes abruptly towards the chemoattractant source. In others (for example, in sea urchin, hydromedusa, fern, or fish such as Japanese bitterlings), the approach to the chemoattractant source is indirect and the movement is by repetitive loops of small radii. In some species (for example, herring or the ascidian Ciona) activation of motility precedes chemotaxis (Cosson, 1990; Kaupp et al., 2006; Miller, 1985; Morisawa, 1994). In chemotaxis, cells may either sense a temporal gradient of the chemoattractant, comparing the occupancy of its receptors at different time points [as do bacteria (Macnab and Koshland, 1972)], or they may detect a spatial gradient, comparing the occupancy of receptors at different locations along the cell [as do leukocytes (Devreotes and Zigmond, 1988)]. In the best-studied species, sea urchin, the spermatozoa sense a temporal gradient (Kaupp et al., 2003) and respond to it with a transient increase in flagellar asymmetry. The outcome is a turn in the swimming path, followed by a period of straight swimming (Kaupp et al., 2003), leading to the observed epicycloid-like movements directed towards the chemoattractant source (Böhmer et al., 2005).
Molecular mechanism
The molecular mechanism of sperm chemotaxis is still not fully known. The current knowledge is mainly based on studies in the sea urchin Arbacia punctulata, where binding of the chemoattractant resact (Table 1) to its receptor, a guanylyl cyclase, activates cGMP synthesis (Figure 1). The resulting rise of cGMP possibly activates K+-selective ion channels. The consequential hyperpolarization activates hyperpolarization-activated and cyclic nucleotide-gated (HCN) channels. The depolarizing inward current through HCN channels possibly activates voltage-activated Ca2+ channels, resulting in elevation of intracellular Ca2+. This rise leads to flagellar asymmetry and, consequently, to a turn of the sperm cell (Kaupp et al., 2006).
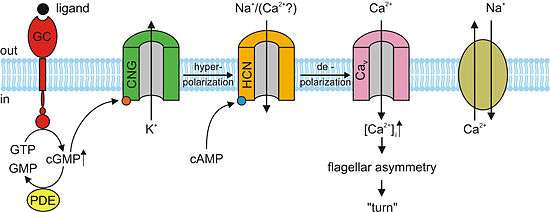
Figure 1. A model of the signal-transduction pathway during sperm chemotaxis of the sea urchin Arbacia punctulata. Binding of a chemoattractant (ligand) to the receptor — a membrane-bound guanylyl cyclase (GC) — activates the synthesis of cGMP from GTP. Cyclic GMP possibly opens cyclic nucleotide-gated (CNG) K+-selective channels, thereby causing hyperpolarization of the membrane. The cGMP signal is terminated by the hydrolysis of cGMP through phosphodiesterase (PDE) activity and inactivation of GC. On hyperpolarization, hyperpolarization-activated and cyclic nucleotide-gated (HCN) channels allow the influx of Na+ that leads to depolarization and thereby causes a rapid Ca2+ entry through voltage-activated Ca2+ channels (Cav), Ca2+ ions interact by unknown mechanisms with the axoneme of the flagellum and cause an increase of the asymmetry of flagellar beat and eventually a turn or bend in the swimming trajectory. Ca2+ is removed from the flagellum by a Na+/Ca2+ exchange mechanism. [Taken from (Kaupp et al., 2006).]
Sperm guidance in mammals: I. Chemotaxis
Following the findings that human spermatozoa accumulate in follicular fluid (Ralt et al., 1991; Villanueva-Díaz et al., 1990) and that there is a remarkable correlation between this in vitro accumulation and oocyte fertilization (Ralt et al., 1991), chemotaxis was substantiated as the cause of this accumulation (Ralt et al., 1994). Sperm chemotaxis was later also demonstrated in mice (Giojalas and Rovasio, 1998; Oliveira et al., 1999) and rabbits (Fabro et al., 2002). In addition, sperm accumulation in follicular fluid (but without substantiating that it truly reflects chemotaxis) was demonstrated in horses (Navarro et al., 1998) and pigs (Serrano et al., 2001). A key feature of sperm chemotaxis in humans is that this process is restricted to capacitated cells (Cohen-Dayag et al., 1995; Eisenbach, 1999) — the only cells that possess the ability to penetrate the oocyte and fertilize it (Jaiswal and Eisenbach, 2002). This raised the possibility that, in mammals, chemotaxis is not solely a guidance mechanism but it is also a mechanism of sperm selection (Cohen-Dayag et al., 1995; Eisenbach, 1999). Importantly, the fraction of capacitated (and, hence, chemotactically responsive) spermatozoa is low (~10% in humans), the life span of the capacitated/chemotactic state is short (1–4 hours in humans), a spermatozoon can be at this state only once in its lifetime, and sperm individuals become capacitated/chemotactic at different time points, resulting in continuous replacement of capacitated/chemotactic cells within the sperm population, i.e., prolonged availability of capacitated cells (Cohen-Dayag et al., 1994; 1995). These sperm features raised the possibility that prolonging the time period, during which capacitated spermatozoa can be found in the female genital tract, is a mechanism, evolved in humans, to compensate for the lack of coordination between insemination and ovulation (Cohen-Dayag et al., 1995; Eisenbach, 1999; Eisenbach and Giojalas, 2006; Giojalas et al., 2004).
Chemoattractants
In humans, there are at least two different origins of sperm chemoattractants. One is the mature oocyte, and the other is the cumulus cells that surround the oocyte (Sun et al., 2005). The identity of the chemoattractant secreted from the oocyte is not known; the one secreted from the cumulus cells is the steroid progesterone (Guidobaldi et al., 2008; Oren-Benaroya et al., 2008; Teves et al., 2006). Additional sperm chemoattractants have been found, examples are atrial natriuretic peptide (ANP) (Zamir et al., 1993) and specific odorants (Spehr et al., 2003) [complete chemoattractant lists can be found in (Eisenbach, 2004; Eisenbach and Giojalas, 2006)], but it is an open question whether they are physiologically involved in guidance in vivo.
Species specificity
Species specificity was not detected in experiments that compared the chemotactic responsiveness of human and rabbit spermatozoa to follicular fluids or egg-conditioned media obtained from human, bovine, and rabbit (Sun et al., 2003). The subsequent findings that cumulus cells of both human and rabbit (and, probably, of other mammals as well) secrete the chemoattractant progesterone (Guidobaldi et al., 2008; Oren-Benaroya et al., 2008; Teves et al., 2006) is sufficient to account for the lack of specificity in the chemotactic response of mammalian spermatozoa.
Behavioral mechanism
Mammalian spermatozoa, like sea-urchin spermatozoa, appear to sense a temporal chemoattractant gradient. This is because the establishment of a temporal gradient in the absence of spatial gradient, achieved by mixing human spermatozoa with a chemoattractant, results in a delayed transient increase in swimming velocity and lateral head displacement, leading to a delayed transient decrease in the linearity of swimming (Gakamsky et al., 2009). When the chemoattractant concentration is high, the spermatozoa exhibit hyperactivated motility. Likewise, photorelease of cyclic nucleotides, considered as putative second messengers of the chemotactic pathway(s) (Kaupp et al., 2003; Spehr et al., 2004), from their caged compounds cause a delayed decrease in the linearity of swimming (Gakamsky et al., 2009), similar to that observed upon the photorelease of progesterone (Kilic et al., 2009). On the basis of these observations it was suggested that the response characteristics observed in temporal gradients also occur in spatial gradients: an excitation phase, composed of a delay (i.e., no change in the motility parameters) and a subsequent turn, and an adaptation phase during which the cell ceases to respond to the chemoattractant even though it is still present. When a spermatozoon swims up a spatial gradient, the turning step is repressed. When it swims down a gradient or in no gradient, the cell turns. In the latter case, if not re-stimulated, the cell eventually adapts and resumes its original swimming mode (Gakamsky et al., 2009).
Molecular mechanism
Very little is known on the molecular mechanism of sperm chemotaxis in mammals. It is even not known whether it is similar to that of sea-urchin spermatozoa because, on the one hand, assuming universality — the molecular mechanisms may be similar. The finding in human spermatozoa that ANP, a known activator of particulate guanylyl cyclase, is chemotactically active and the consequent suggestion that ANP may directly affect guanylyl cyclase in a manner similar to that caused by the physiological attractant (Zamir et al., 1993) are in line with this possibility. On the other hand, the identification of the odorant receptor hOR17-4 on human spermatozoa and the demonstration of sperm chemotaxis to its agonist bourgeonal (Spehr et al., 2003) suggest that mammalian sperm chemotaxis involves a signal transduction pathway similar to that of the olfactory system. This may seem a valid possibility in view of the finding that male germ cells appear to contain all the elements of the signaling cascade present in olfactory cells (Defer et al., 1998) and the observation that bourgeonal induces a transient Ca2+ influx in about one third of the cells of human spermatozoa, a response that is inhibited by an adenylyl cyclase inhibitor (Spehr et al., 2003; Spehr et al., 2004). It is possible that mammalian spermatozoa possess both signal-transduction systems. The observation that intracellular photorelease of each cAMP and cGMP causes a similar behavioral response (Gakamsky et al., 2009) is consistent with this possibility.
Sperm guidance in mammals: II. Thermotaxis
At ovulation, at least in rabbits (Bahat et al., 2005; David et al., 1972) and pigs (Hunter and Nichol, 1986), a temperature difference of 1–2 °C is established within the oviduct, the temperature being higher at the fertilization site than at the junction between the uterus and the oviduct, close to the sperm storage site in the oviduct (Suarez, 2002). This difference is formed by a time-dependent temperature drop at the uterus-oviduct junction, a drop that occurs in spite of the simultaneous rise in body temperature at ovulation (Bahat et al., 2005). Following the suggestion of Hunter (1998) that this temperature difference might serve as a cue for guiding spermatozoa to the site of fertilization, Bahat et al. (2003) demonstrated that rabbit and human spermatozoa are able to sense small temperature differences and respond to them by thermotaxis. The temperature gradient in the rabbit oviduct was calculated to be at the order of 0.1 °C/cm (Bahat and Eisenbach, 2006), within the range of known thermotaxis systems. As in sperm chemotaxis, only capacitated spermatozoa are thermotactically responsive (Bahat et al., 2003).
Sperm guidance in mammals: III. Chemotaxis and thermotaxis combined
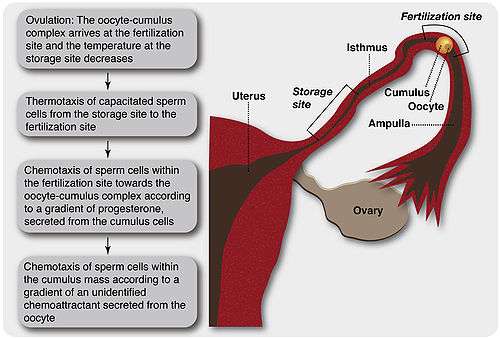
The findings that mammalian spermatozoa are capable to respond to a chemoattractant gradient by chemotaxis and to a temperature gradient by thermotaxis and that both such gradients are established in the female genital tract raised the possibility that spermatozoa guidance is done by both chemotaxis and thermotaxis. It was further suggested that, in vivo, each mechanism is functional in a region of the oviduct where the other mechanism is ineffective (Bahat et al., 2003; Eisenbach and Giojalas, 2006). According to this suggestion (Figure 2), capacitated spermatozoa, released from the sperm storage site at the isthmus (Suarez, 2002), may be first guided by thermotaxis from the cooler sperm storage site towards the warmer fertilization site (Bahat et al., 2003). Passive contractions of the oviduct (Battalia and Yanagimachi, 1979) may assist the spermatozoa to reach there. At this location the spermatozoa may be chemotactically guided to the oocyte-cumulus complex by the gradient of progesterone, secreted from the cumulus cells. In addition, progesterone may inwardly guide spermatozoa, already present within the periphery of the cumulus oophorus (Teves et al., 2006). Spermatozoa that are already deep within the cumulus oophorus may sense the more potent chemoattractant that is secreted from the oocyte (Sun et al., 2005) and chemotactically guide themselves to the oocyte according to the gradient of this chemoattractant. It should be borne in mind, however, that this is only a model. In view of the increasing number of different chemoattractants that are being discovered, the guidance in vivo might be much more complex.
Figure 2. A simplified scheme describing the suggested sequence of sperm guidance events in mammals.
Potential clinical applications
Sperm guidance by either chemotaxis or thermotaxis can potentially be used to obtain sperm populations that are enriched with capacitated spermatozoa for in vitro fertilization procedures. They can also be exploited as a diagnostic tool to assess sperm quality. In addition, these processes can potentially be used, in the long run, as a means of contraception by interfering with the normal process of fertilization (Eisenbach and Tur-Kaspa, 1999; Eisenbach and Giojalas, 2006).
References
- Al-Anzi, B. and Chandler, D.E. (1998). "A sperm chemoattractant is released from Xenopus egg jelly during spawning." Dev. Biol. 198, 366–375.
- Bahat, A., Tur-Kaspa, I., Gakamsky, A., Giojalas, L.C., Breitbart, H. and Eisenbach, M. (2003). "Thermotaxis of mammalian sperm cells: A potential navigation mechanism in the female genital tract". Nature Med. 9, 149–150.
- Bahat, A., Eisenbach, M. and Tur-Kaspa, I. (2005). "Periovulatory increase in temperature difference within the rabbit oviduct". Hum. Reprod. 20, 2118–2121.
- Bahat, A. and Eisenbach, M. (2006). "Sperm thermotaxis". Mol. Cell. Endocrinol. 252, 115–119.
- Battalia, D.E. and Yanagimachi, R. (1979). "Enhanced and co-ordinated movement of the hamster oviduct during the periovulatory period". J. Reprod. Fert. 56, 515–520.
- Böhmer, M., Van, Q., Weyand, I., Hagen, V., Beyermann, M., Matsumoto, M., Hoshi, M., Hildebrand, E. and Kaupp, U.B. (2005). "Ca2+ spikes in the flagellum control chemotactic behavior of sperm". EMBO J. 24, 2741–2752.
- Brokaw, C.J. (1958). "Chemotaxis of bracken spermatozoids. The role of bimalate ions". J. Exp. Zool. 35, 192–196.
- Cohen-Dayag, A., Ralt, D., Tur-Kaspa, I., Manor, M., Makler, A., Dor, J., Mashiach, S. and Eisenbach, M. (1994). "Sequential acquisition of chemotactic responsiveness by human spermatozoa". Biol. Reprod. 50, 786–790.
- Cohen-Dayag, A., Tur-Kaspa, I., Dor, J., Mashiach, S. and Eisenbach, M. (1995). "Sperm capacitation in humans is transient and correlates with chemotactic responsiveness to follicular factors". Proc. Natl. Acad. Sci. U.S.A. 92, 11039–11043.
- Coll, J.C. and Miller, R.L. (1992). "The nature of sperm chemo-attractants in coral and starfish". In: Comparative Spermatology: 20 Years After (Baccetti, B., ed.) pp. 129–134. Raven Press, New York.
- Cosson, M.P. (1990). "Sperm chemotaxis". In: Controls of Sperm Motility: Biological and Clinical Aspects (Gagnon, C., ed.) pp. 103–135. CRC Press, Boca Raton, FL.
- David, A., Vilensky, A. and Nathan, H. (1972). "Temperature changes in the different parts of the rabbit's oviduct". Int. J. Gynaec. Obstet. 10, 52–56.
- Defer, N., Marinx, O., Poyard, M., Lienard, M.O., Jegou, B. and Hanoune, J. (1998). "The olfactory adenylyl cyclase type 3 is expressed in male germ cells". FEBS Lett. 424, 216–220.
- Devreotes, P.N. and Zigmond, S.H. (1988). "Chemotaxis in eukaryotic cells: a focus on leukocytes and Dictyostelium". Annu Rev Cell Biol 4, 649–86.
- Eisenbach, M. and Tur-Kaspa, I. (1994). "Human sperm chemotaxis is not enigmatic anymore". Fertil. Steril. 62, 233–235.
- Eisenbach, M. (1999). "Mammalian sperm chemotaxis and its association with capacitation". Dev. Genet. 25, 87–94.
- Eisenbach, M. and Tur-Kaspa, I. (1999). "Do human eggs attract spermatozoa?" BioEssays 21, 203–210.
- Eisenbach, M. (2004). "Chemotaxis." Imperial College Press, London.
- Eisenbach, M. and Giojalas, L.C. (2006). "Sperm guidance in mammals - an unpaved road to the egg." Nature Rev. Mol. Cell Biol. 7, 276–285.
- Fabro, G., Rovasio, R.A., Civalero, S., Frenkel, A., Caplan, S.R., Eisenbach, M. and Giojalas, L.C. (2002). "Chemotaxis of capacitated rabbit spermatozoa to follicular fluid revealed by a novel directionality-based assay". Biol. Reprod. 67, 1565–1571.
- Gakamsky, A., Armon, L. and Eisenbach, M. (2009). "Behavioral response of human spermatozoa to a concentration jump of chemoattractants or intracellular cyclic nucleotides." Hum. Reprod. 24, In press.
- Giojalas, L.C. and Rovasio, R.A. (1998). "Mouse spermatozoa modify their dynamic parameters and chemotactic response to factors from the oocyte microenvironment". Int. J. Androl. 21, 201–206.
- Giojalas, L.C., Rovasio, R.A., Fabro, G., Gakamsky, A. and Eisenbach, M. (2004). "Timing of sperm capacitation appears to be programmed according to egg availability in the female genital tract". Fertil. Steril. 82, 247–249.
- Guidobaldi, H.A., Teves, M.E., Unates, D.R., Anastasia, A. and Giojalas, L.C. (2008). "Progesterone from the cumulus cells is the sperm chemoattractant secreted by the rabbit oocyte cumulus complex". PLoS ONE 3, e3040.
- Hunter, R.H.F. and Nichol, R. (1986). "A preovulatory temperature gradient between the isthmus and the ampulla of pig oviducts during the phase of sperm storage". J. Reprod. Fert. 77, 599–606.
- Hunter, R.H.F. (1998). "Sperm-epithelial interactions in the isthmus and ampulla of the Fallopian tubes and their ovarian control". In: Gamettes: Development and Function (Lauria, A., Gandolfi, F., Enne, G. and Gianaroli, L., eds.), pp. 355–367. Serono Symposia, Rome.
- Jaiswal, B.S. and Eisenbach, M. (2002). "Capacitation". In: Fertilization (Hardy, D.M., ed.) pp. 57–117. Academic Press, San Diego.
- Kaupp, U.B., Solzin, J., Hildebrand, E., Brown, J.E., Helbig, A., Hagen, V., Beyermann, M., Pampaloni, F. and Weyand, I. (2003). "The signal flow and motor response controlling chemotaxis of sea urchin sperm". Nature Cell Biol. 5, 109–117.
- Kaupp, U.B., Hildebrand, E. and Weyand, I. (2006). "Sperm chemotaxis in marine invertebrates - molecules and mechanism." J. Cell. Physiol. 208, 487–494.
- Kilic, F., Kashikar, N.D., Schmidt, R., Alvarez, L., Dai, L., Weyand, I., Wiesner, B., Goodwin, N., Hagen, V. and Kaupp, U.B. (2009). "Caged progesterone: a new tool for studying rapid nongenomic actions of progesterone." J. Am. Chem. Soc. 131, 4027–4030.
- Macnab, R.M. and Koshland, D.E. (1972). "The gradient-sensing mechanism in bacterial chemotaxis." Proc. Natl. Acad. Sci. U.S.A. 69, 2509–2512.
- Maier, I. and Müller, D.G. (1986). "Sexual pheromones in algae'. Biol. Bull. 170, 145–175.
- Miller, R.D. and Vogt, R. (1996). "An N-terminal partial sequence of the 13kDa Pycnopodia helianthoides sperm chemoattractant 'startrak' possesses sperm-attracting activity". J. Exp. Biol. 199, 311–318.
- Miller, R.L. (1985). "Sperm chemo-orientation in the metazoa". In: Biology of Fertilization (Metz, C.B. and Monroy, A., eds.), pp. 275–337. Academic Press, New York.
- Miller, R.L. (1997). "Specificity of sperm chemotaxis among great barrier reef shallow-water holothurians and ophiuroids". J. Exp. Zool. 279, 189–200.
- Morisawa, M. (1994). "Cell signaling mechanisms for sperm motility". Zool. Sci. 11, 647–662.
- Navarro, M.C., Valencia, J., Vazquez, C., Cozar, E. and Villanueva, C. (1998). "Crude mare follicular fluid exerts chemotactic effects on stallion spermatozoa". Reprod. Domest. Anim. 33, 321–324.
- Oliveira, R.G., Tomasi, L., Rovasio, R.A. and Giojalas, L.C. (1999). "Increased velocity and induction of chemotactic response in mouse spermatozoa by follicular and oviductal fluids". J. Reprod. Fertil. 115, 23–27.
- Olson, J.H., Xiang, X.Y., Ziegert, T., Kittelson, A., Rawls, A., Bieber, A.L. and Chandler, D.E. (2001). "Allurin, a 21-kDa sperm chemoattractant from Xenopus egg jelly, is related to mammalian sperm-binding proteins". Proc. Natl. Acad. Sci. U.S.A. 98, 11205–11210.
- Oren-Benaroya, R., Orvieto, R., Gakamsky, A., Pinchasov, M. and Eisenbach, M. (2008). "The sperm chemoattractant secreted from human cumulus cells is progesterone". Hum. Reprod. 23, 2339–2345.
- Pfeffer, W. (1884). "Lokomotorische richtungsbewegungen durch chemische reize". Untersuch. aus d. Botan. Inst. Tübingen 1, 363–482.
- Poff, K.L. and Skokut, M. (1977). "Thermotaxis by pseudoplasmodia of Dictyostelium discoideum". Proc. Natl. Acad. Sci. U.S.A. 74, 2007–2010.
- Ralt, D., Goldenberg, M., Fetterolf, P., Thompson, D., Dor, J., Mashiach, S., Garbers, D.L. and Eisenbach, M. (1991). "Sperm attraction to a follicular factor(s) correlates with human egg fertilizability". Proc. Natl. Acad. Sci. U.S.A. 88, 2840–2844.
- Ralt, D., Manor, M., Cohen-Dayag, A., Tur-Kaspa, I., Makler, A., Yuli, I., Dor, J., Blumberg, S., Mashiach, S. and Eisenbach, M. (1994). "Chemotaxis and chemokinesis of human spermatozoa to follicular factors". Biol. Reprod. 50, 774–785.
- Serrano, H., Canchola, E. and García-Suárez, M.D. (2001). "Sperm-attracting activity in follicular fluid associated to an 8.6-kDa protein". Biochem. Biophys. Res. Commun. 283, 782–784.
- Spehr, M., Gisselmann, G., Poplawski, A., Riffell, J.A., Wetzel, C.H., Zimmer, R.K. and Hatt, H. (2003). "Identification of a testicular odorant receptor mediating human sperm chemotaxis". Science 299, 2054–2058.
- Spehr, M., Schwane, K., Riffell, J.A., Barbour, J., Zimmer, R.K., Neuhaus, E.M. and Hatt, H. (2004). "Particulate adenylate cyclase plays a key role in human sperm olfactory receptor-mediated chemotaxis". J. Biol. Chem. 279, 40194–40203.
- Starr, R.C., Marner, F.J. and Jaenicke, L. (1995). "Chemoattraction of male gametes by a pheromone produced by female gametes of chlamydomonas". Proc. Natl. Acad. Sci. U.S.A. 92, 641–645.
- Suarez, S.S. (2002). "Gamete transport". In: Fertilization (Hardy, D.M., ed.) pp. 3–28. Academic Press, San Diego.
- Sun, F., Giojalas, L.C., Rovasio, R.A., Tur-Kaspa, I., Sanchez, R. and Eisenbach, M. (2003). "Lack of species-specificity in mammalian sperm chemotaxis". Dev. Biol. 255, 423–427.
- Sun, F., Bahat, A., Gakamsky, A., Girsh, E., Katz, N., Giojalas, L.C., Tur-Kaspa, I. and Eisenbach, M. (2005). "Human sperm chemotaxis: both the oocyte and its surrounding cumulus cells secrete sperm chemoattractants". Hum. Reprod. 20, 761–767.
- Teves, M.E., Barbano, F., Guidobaldi, H.A., Sanchez, R., Miska, W. and Giojalas, L.C. (2006). "Progesterone at the picomolar range is a chemoattractant for mammalian spermatozoa". Fertil. Steril. 86, 745–749.
- Villanueva-Díaz, C., Vadillo-Ortega, F., Kably-Ambe, A., Diaz-Perez, M.A. and Krivitzky, S.K. (1990). "Evidence that human follicular fluid contains a chemoattractant for spermatozoa". Fertil. Steril. 54, 1180–1182.
- Ward, G.E., Brokaw, C.J., Garbers, D.L. and Vacquier, V.D. (1985). "Chemotaxis of Arbacia punctulata spermatozoa to resact, a peptide from the egg jelly layer". J. Cell Biol. 101, 2324–2329.
- Yoshida, M., Inaba, K. and Morisawa, M. (1993). "Sperm chemotaxis during the process of fertilization in the ascidians Ciona-Savignyi and Ciona-Intestinalis". Dev. Biol. 157, 497–506.
- Yoshida, M., Inaba, K., Ishida, K. and Morisawa, M. (1994). "Calcium and cyclic AMP mediate sperm activation, but Ca2+ alone contributes sperm chemotaxis in the ascidian, Ciona savignyi". Dev. Growth Dif. 36, 589–595.
- Yoshida, M., Murata, M., Inaba, K. and Morisawa, M. (2002). "A chemoattractant for ascidian spermatozoa is a sulfated steroid". Proc. Natl. Acad. Sci. U.S.A. 99, 14831–14836.
- Zamir, N., Riven-Kreitman, R., Manor, M., Makler, A., Blumberg, S., Ralt, D. and Eisenbach, M. (1993). "Atrial natriuretic peptide attracts human spermatozoa in vitro". Biochem. Biophys. Res. Commun. 197, 116–122.
- Zatylny, C., Marvin, L., Gagnon, J. and Henry, J.L. (2002). "Fertilization in Sepia officinalis: the first mollusk sperm-attracting peptide". Biochem. Biophys. Res. Commun. 296, 1186–1193.